Entropy Quantum Computing Offers an Innovative Path Toward Scalable Quantum Computing
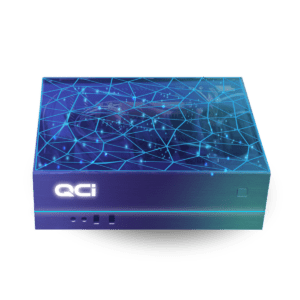
For most scientists – and especially those physicists exploring quantum information science – the mere mention of words like “entropy” and “decoherence” are enough to keep them up at night.
The research team at Quantum Computing Inc. – QCi – does not suffer from scientific term-induced insomnia. In fact, they see entropy and decoherence as keys to an albeit contrarian, but rigorously scientifically tested, path to practical quantum computing.
In fact, QCi made entropy the first name in one of its latest quantum technologies, Entropy Quantum Computing. The technology is the engineering marvel behind Dirac 1, the company’s quantum photonic computing system.
Most current quantum computing architectures operate on the premise that noise is bad. These architectures prefer to operate in pristine environments with little noise so that their qubits can perform calculations with little interference. Just a little noise can collapse quantum states and throw those computations off.
It’s easier said than done. These systems, often referred to as closed quantum systems, require extreme engineering to calm those noisy effects and minimize interactions with the environment. Extreme engineering is expensive engineering. Huge, energy-draining cryostatic devices and deviously precise wiring are required in most of these set-ups – and those are just some of the expensive engineering challenges that plague closed systems.
For domain experts seeking answers to the world’s most challenging problems, closed quantum systems can add another layer of issues and difficulties.
Open Quantum Systems
EQC photonic system, on the other hand, is an open quantum system. In this system, a quantum system rests within an engineered environment so that when its quantum state collapses, it fits the solution to the designated problem. In an open system, the engineering requirements are kept to a minimum. The system can operate at room temperature, for instance, and support computations over a many-variable space.
Unlike closed quantum systems, the environment is a free source of useful energy. Using the effect of interaction with the environment – called backaction in quantum terms – to evolve the quantum system into a space that is decoherence-free. This sub-space is not susceptible to the effects of decoherence.
QCi’s Chief Technology Officer and Chief Operating Officer William McGann explains that while most see entropy as noise, QCi researchers see it as potential.
“People call it noise, or they refer to entropy as chaotic energy, because it doesn’t do useful work,” said McGann. “What we’ve done is we have conditioned the entropy to do useful work, that’s essentially what we have accomplished.
He added, “The only difference between energy and entropy is chaos. One is undirected. To use an analogy, you could say that it’s like the difference between emotion and passion in humans. Passion is directed emotions. So, we create an engineered noise environment that we put in our local system. And we do that by measurement and introducing bias as needed. Although, sometimes you don’t even need to do anything. The noise can be white, meaning that they are uniform in spectrum. Or it can be colorful, with memory effects and non-Markovian characteristics. When we know we have a non-Markovian situation, we turn our oscillator on and then it’s simply programming a function. For example, you program entropy in mode one or couple this much entropy in mode two. In that case, what we have done is added energy to each of those modes differently now.”
Each of those modes has a different wave function and these modes no longer degenerate, said McGann.
“They all are at a different energy level, because I’ve coupled different amounts of the entropy to them,” he said. “In an optical line, there can be thousands of these modes, so, essentially, there could be thousands of effective qubits. This setup is just with an oscillator, a laser and fiber optics.”
McGann said that the system is not trivial to engineer or trivial to couple, but through proper engineering, QCi’s research team – like one of our favorite fairy tale porridge samplers – can find just the right balance among all the complex variables that go into prompting usable entropy quantum computations.
“It’s a Goldilocks thing where it’s collapsed, but it’s stable,” said McGann. “If you don’t couple it with the right energy, the qubits will collapse. If you couple it at what they call the Rabi frequency, you’ll have a coherent state. If you overcomplicate it, it will collapse even faster, which is called the sudden death of entanglement theory. But, that said, you can create coherence in these modes.”
Radioactive decay is, loosely, a similar system in the natural world.
In essence, the system resembles a quantum harmonic oscillator with photons, rather than electrons. Well-known in quantum science, the oscillator is a distinct upside-down parabola striped with horizontal lines that cross the well and tighten as the lines move closer to the top.
“This forms a ladder – and the ladder is energy, which gets closer and closer,” said McGann. “That’s true for our system, too. As you get farther and farther, the greater the number of qudits or qubits we create. They start to compress until you run into the limit where you can’t tell one level from another.
The team estimates that the current limit is about 30,000 qubits, but that could expand as research progresses.
Quantum Zeno Effect
EQC also relies on the quantum Zeno effect, a well noted phenomenon in the quantum world. The Zeno effect – which couples Greek philosopher’s Zeno of Elea’s paradox on continuity with a quantum version “watched pot never boils” – describes how unstable particles if observed continuously never decay.
For EQC that bizarre state is actually a door to a back door to coherence.
“If I measure something frequently enough, I basically freeze it in time,” said McGann. “And so we find that, at some point, you start measuring so fast that literally time stops, it freezes. That’s possible to do in the quantum world by how you excite quantum states, which is called the Quantum Zeno Effect. When you do that, you create what they call decoherent free space. But it’s actually coherence.”
Qudits
EQC technology also lends itself to the use of quantum digits – or qudits – according to McGann. A qudit is similar to a qubit, except qudits have a different dimension greater than two. Qudits could conceivably be even more powerful for EQC because qudits provide a larger state space to store and process information
“In fact, it may be easier for us to build a qudit machine than a qubit machine because what we have to do to build a qubit is manually restrict the number of available modes for each photon to be two, which could cause errors” said McGann
Simplicity
Contrarian does not mean unnecessarily complex. In fact, EQC’s real advantage is that that the essence of EQC’s effectiveness – and its ability to perform in normal environments – is in the system’s simplicity, especially when compared to other more elaborate approaches to quantum computing.
The basic set-up is not only one of the reasons that QCi has great hope in EQC’s ability to bring real world solutions by operating in normal environments, but it also points to a future of continual improvements and upgrades.
It Works
Even though the system is, comparatively, in its infancy, EQC is already showing results tackling some tricky real-world optimization problems for companies. For example, the company helped BMW find the correct placement of sensors on a car, which could one day help the automotive leader design autonomous vehicles.
According to McGann, the problem was complex with BMW listing more than 500 constraints that would affect sensor placement.
“Some of those constraints were fairly obvious, such as not projecting the LIDAR sensors from the passenger’s side to the driver’s side because light doesn’t go through the car, but they’re also important constraints, such as limiting the total of more than three types of sensors.”
Even with those constraints, EQC only needed about six minutes to arrive at the optimal solution for sensor placement. The solution would allow BMW to strategically place 15 sensors to cover about 97 percent of the vehicle.
“EQC operates on an Ising formulation of a Hamiltonian,” according to a company statement. “By stating the sensor placement problem as a Hamiltonian, we can use the Ising formulation to find a ground state of the system through EQC. There are no circuits to build. Once the Ising formulation is built, the computer can accept the full problem definition and get to work on finding the ground state.”
The possibilities of how EQC could be used “in the wild” is not limited to just sensor placement. Optimization problems vex many businesses and organizations. McGann could see EQC providing solutions – right now – to challenges that affect the climate, such as the proper placement of wind turbines for maximum energy creation and efficiency. He said communication companies could use it to determine where to place cellular phone towers to offer customers the best coverage possible.
Tests of EQC for this problem included problems of size 1,023, 2,888 and 3,854 in terms of variable count. Once we confirmed our initial runs of the 3,854-variable problem, we only tested it. Unless noted otherwise, results stated here are for that problem.
“We just said, program the Hamiltonian, put it in and turn it on. And we got an answer in six minutes,” said McGann.
McGann said that the technology has showed incredible performance even though it’s relatively new. The team is looking forward to the next steps to expand and enhance the technology, particularly in miniaturizing components, which will bring EQC into the real world where it can be leveraged to solve difficult computational challenges around the world.